Researchers create hybrids of six yeast species to combine useful traits
Researchers at the Great Lakes Bioenergy Research Center at the University of Wisconsin–Madison have developed a method to combine traits from up to six different yeast species in a single hybrid strain — a yeast that could carry more tools for a specific job, such as producing biofuels.
“This technology that we developed is a way to try to combine the best traits of different species when you don’t know exactly where these are encoded in the genome,” says researcher David Peris, who led the work as a postdoctoral fellow at UW–Madison and a Marie Sklodowska Curie fellow at the Institute of Agrochemistry and Food Technology-CSIC in Spain.
The idea, he says, is to combine as many genomes as possible to maximize the amount of genetic starting material in which to look for promising characteristics. Then the researchers can expose the resulting gene-rich hybrids to various conditions in the laboratory to identify strains that express a desired trait, such as the ability to grow at cold temperatures or to digest plant sugars.
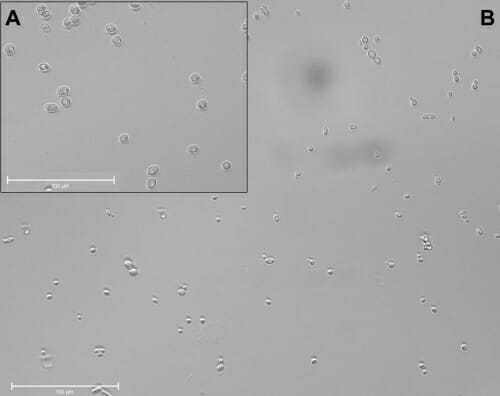
Microscope images of Saccharomyces cerevisiae yeast cells compare new six-species hybrids (in panel A) to parent yeast cells (in panel B). Both images are at the same scale, showing how the size of the new hybrids increased compared to the parents. Image courtesy Amanda Hulfachor, David Peris and Kaitlin Fisher
With their new method, called iterative hybrid production (iHyPr), the team successfully produced hybrid yeasts from six different Saccharomyces species. The multi-species hybrids adapt quickly to their environments, making them well suited to laboratory-based evolutionary selection.
“It’s very common to cross different organisms with desirable properties,” such as different varieties of corn or breeds of dogs, says senior author Chris Hittinger, a UW–Madison genetics professor. “What’s different here is that we’re crossing things that are different species, and are quite distinct genetically.”
Two-species hybrids are widely used in agriculture, food and beverage production and other industries. For example, lager beer is produced by a two-species hybrid yeast. A handful of three- and four-species hybrids are known in the beverage industry, Hittinger says, but it isn’t clear how they developed.
The iHyPr method essentially hijacks the yeast’s mating process to trick the organisms into forming higher-order hybrids.
“It really was to see how far we could push the yeast mating system to create as complex of hybrids as possible,” Hittinger says.
Hittinger sees many potential research and industrial uses for such hybrids, including studies in genetics, cancer biology, brewing and bioenergy.
Yeast cells have two mating type genes, “a” and “alpha.” Normally, only cells with a single set of chromosomes, called haploid cells, can mate; they carry a single mating gene, either “a” or “alpha.” They seek out haploid cells expressing the other mating gene and combine to form a diploid organism with two sets of chromosomes, one from each haploid cell. And normally, that’s it.
But the team used an enzyme that can change one mating-type gene into the other. By changing some a-type cells into alpha-type, the team was able to make crosses that create a mating-ready cell that has two complete sets of chromosomes. Two of these diploid cells from different species could then be mated to create a two-species hybrid with four sets of chromosomes.
“Basically, we were hacking the biological system for facilitating crosses of diploids,” Peris explains.
In the new study, the researchers described a way to iterate the method to create even more complex hybrids. So far, they’ve been able to combine the genomes of six yeast species into a single cell. The work was published April 29 in Nature Communications.
“One of the reasons this works is there’s no chromosome counting mechanism in the cell,” Hittinger says. “All it’s doing is looking at whether ‘a’ and ‘alpha’ are both present or not. Even if it has six copies of “a,” say you could find another cell that has six copies of “alpha,” then they’ll mate.”
He isn’t sure if it will be possible to push beyond six species, noting that cell size and chromosome instability both increase with more genomes.
Combining the method with gene editing tools, such as the CRISPR-Cas9 system, opens the door to creating complex hybrid yeasts with specific sets of desired traits. Hittinger sees many potential research and industrial uses for such hybrids, including studies in genetics, cancer biology, brewing and bioenergy. The Wisconsin Alumni Research Foundation has filed a patent on the new process.
The team is currently working to produce a hybrid yeast with multiple traits to improve the ability to turn plant material into advanced biofuels, such as isobutanol. One parent yeast is a GLBRC-designed strain engineered with genes that enable it to consume xylose, a sugar found in high levels in biomass but which cannot easily be used by most Saccharomyces strains. But the process of pretreating plant material to prepare it for fermentation can produce toxins that many yeast strains cannot tolerate. With iHyPr, the researchers crossed the xylose-consuming strain with others that are able to grow better under the pretreatment conditions, as well as a strain that can produce high levels of isobutanol.
“Through laboratory evolution, we were able to push complex hybrids in the direction we wanted to go for industrially relevant traits,” Hittinger says. “This is a new technique for synthetic biology for combining genomes. It’s an exciting development that I think researchers could take in a lot of directions.”
Additional researchers on the study include current and former Hittinger lab researchers William G. Alexander, who made the first three-species hybrid with the technology; Ryan V. Moriarty, who created the first six-species hybrid; and Russell L. Wrobel, who developed most of the iHyPr parameters and crossing schemes.
This work was supported by the DOE Great Lakes Bioenergy Research Center (DOE BER Office of Science DE-SC0018409 and DE-FC02-07ER64494); National Science Foundation (DEB-1253634); USDA National Institute of Food and Agriculture (Hatch Project 1020204); and the Robert Draper Technology Innovation Fund from the Wisconsin Alumni Research Foundation.